Valve, Heal Thyself: Pioneers Seek Tissue-Engineered Fix for Faulty, Failing Cardiac Parts
Newly built, living heart structures may improve treatment durability and, for children, enable fewer repeat procedures.
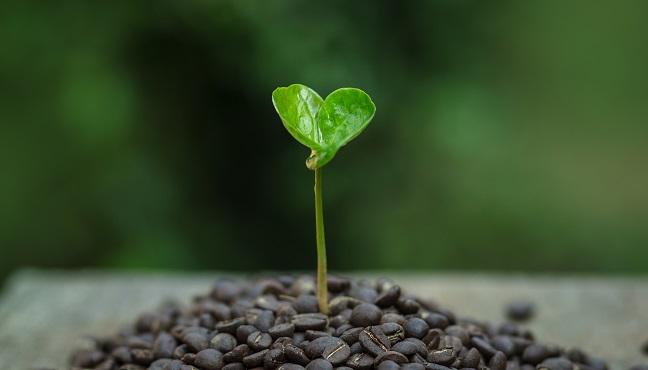
Picture the child, 3 years old, who’s already undergone multiple heart surgeries and faces a lifetime of risky interventions into adulthood. Or the middle-aged TAVR patient who, after 10 good years with a bioprosthetic device, has it begin to fail. What if, rather than relying on artificial prostheses, it was possible to build structures from living tissue?
Being able to grow valves and other heart structures—either by creating synthetic scaffolds that eventually transform or harvesting the raw materials from patients or donors—could potentially alter the course of cardiovascular medicine, experts say.
There are several ways of taking on the challenge of creating products that can heal and grow: implanting homografts taken from the patient’s body, allografts from another human, and xenografts from other species. Grafts can be decellularized and seeded with new cells, or synthetic materials that encourage regeneration can be engineered from scratch.
“This is a big landscape [with] a lot of effort and things going on,” Maximilian Y. Emmert, MD, PhD (University of Zurich, Switzerland), commented to TCTMD. “At the end, it’s not about which is the better one, but which is the best one for the individual patient.”
The recent EuroPCR 2017 meeting in Paris, France, had a session dedicated to the potential advantages and pitfalls of “bioresorbable valves” that start out as cell-free, synthetic-polymer scaffolds and are gradually replaced by the patient’s own cells. The promise here is that, by being more biocompatible, the valves won’t fall prey to the early inflammatory response thought to imperil durability.
“The aim is to guide the body to restoring itself,” Martijn Cox, PhD, chief technology officer and cofounder of Xeltis (Zurich, Switzerland), said in his presentation on endogenous tissue restoration (ETR).
With ETR, the “shape can be whatever you want it to be—it can be a tube or it can be a heart valve,” said Martin B. Leon, MD (NewYork-Presbyterian/Columbia University Medical Center, New York, NY). “And it has mechanical properties that are relatively similar to biological materials. Once you implant it in the body it immediately begins to function as a biologic material, but it is porous and because [it contains a lot of signaling proteins] it attracts a whole variety of endogenous cells.”
Over a period of months and, depending on the anatomic location, perhaps years, the scaffold gets populated by all of these cells and the polymer begins to resorb, he said. “As it bioresorbs, the endogenous cells form literally not just cells but a differentiated tissue, with tissue function. So you’ve now replaced what is a bioresorbable, polymeric scaffold [with] what is now living tissue of the patients’ own cells.”
Sharing his gut reaction to hearing preclinical data on Xeltis’ aortic valve, Leon described himself as being “simply struck that this is pretty wild, it’s pretty crazy, but it’s the kind of thing where you could imagine that with some intense work and proper funding, there’s a chance that this could be a viable and meaningful technology.”
Going Commercial
Xeltis, sources told TCTMD, is the furthest along commercially and clinically of the various cardiovascular tissue-engineering projects under development around the world. “This is the one that’s really caught on, and it’s really taken off into some early-stage clinical trials,” Leon commented.
Xeltis is a “spinoff” of the work done at the Eindhoven University of Technology, said A. Pieter Kappetein, MD, PhD (Erasmus Medical Center, Rotterdam, the Netherlands). “It’s a company now, which really can attract money from investors.”
To TCTMD, Kappetein described ETR as “very promising.” He, too, is active in the area of tissue engineering. “We are currently working on a little bit different concept and building on what Xeltis is doing right now,” Kappetein explained. “We are trying to identify what the right shape is [for] the leaflets, what other material you can use so you don’t get leaflet retraction.” This “more fundamental research,” he said, “could ultimately also be integrated into Xeltis products or other heart valves.”
Growing tissue in situ, as done with ETR, has advantages over techniques in which cells are seeded onto devices outside the body, he said. With these, there are questions over how to keep the device from being contaminated, how to sterilize it, and how to store it, Kappetein noted. “The Xeltis principle is you have something you can [use] off the shelf in the OR, and when the patient needs it you can implant it.”
But Emmert, who recently co-authored a preclinical study on a bioresorbable pulmonary valve published in Biomaterials, pointed out that with the polymer-based approach, there is no guarantee that the valve will regenerate as hoped.
“[It] truly and completely relies on the in situ regeneration of the recipient. So there is no matrix that is supporting the function in the beginning,” he said. While children have very high regenerative potential, adults tend to have more comorbidities and other factors that could limit the ability to regrow tissue. This could prove problematic, Emmert suggested. “When you then put, for example, a fully synthetic-polymer heart valve into the body and it degrades but in parallel to that fails to form the sufficient tissue needed, you will have a functional problem.”
One solution is to grow the necessary structure outside of the body before implantation. This can be done by seeding a foundation that’s either a synthetic valve (what Emmert called “the Zurich approach”) or a graft (“the Hannover approach,” being studied in the ESPOIR trial) with cells harvested from the individual patient or from, for example, umbilical cord tissue. With this technique, the extracellular matrix already exists “and provides more stability and then more safety,” he explained.
To avoid an immunoreaction, the scaffold is decellularized (ie, stripped of the cells that initially grew it), leaving only the extracellular matrix behind. “That is the network that has been built up by the cells,” Emmert said, adding, “By removing the cells, we remove the DNA. And the DNA is what [ultimately] is causing the immunoreaction.”
Other valve-related work is being done at Harvard University and elsewhere, he noted. Moreover, tissue engineering extends beyond the heart, Emmert said, citing the success of grafts for dialysis access in end-stage kidney disease.
Right vs Left
Importantly, when it comes to engineering tissue, the needs differ for the right side and the left side of the heart, Kappetein emphasized. Over the past 15 years, the flurry of innovation into less-invasive approaches to structural heart disease has focused on the left-heart aortic and mitral valves.
Clinical data on the Xeltis technology, however, thus far involves the right position where, Kappetein observed, “the blood pressure is much lower than on the left side. And on the right side of the heart, there are not that many patients who need an intervention. So the number of patients that ultimately would benefit from such a treatment is limited, but [those who will] could benefit from it very well.”
Leon had a slightly different take, noting that the pulmonary valve is “the one with the greatest need, because these are the children that are going to be growing. And the opportunity to be able to allow them to avoid further surgeries would be extraordinary.”
According to a Xeltis press release, approximately 100,000 children are born each year with life-threatening congenital heart defects that require pulmonary valve replacement. By contrast, the number of annual TAVR cases is predicted to reach 289,000 worldwide by 2025.
Ethically, when are you ready to implant [a new device] into a human being? A. Pieter Kappetein
Two-year data on the company’s vascular graft for pediatric patients were recently published in the Journal of Thoracic and Cardiovascular Surgery. Xplore-I, the first feasibility clinical trial for Xeltis’ pulmonary valve, is underway in Europe and Asia, where 12 children were successfully implanted with the device as of December 2016. This January, the US Food and Drug Administration gave the go-ahead to Xplore-II, which will test the pulmonary valve in 10 pediatric patients at four centers in the United States.
Kappetein pointed out that there are special considerations when it comes to pediatric patients, asking, “Ethically, when are you ready to implant [a new device] into a human being?”
Novel technologies are oftentimes first studied in regions of the world that have fewer restrictions on research, and this can be problematic, he observed. “It’s children, and of course sometimes people use the argument of, ‘If we did not use this new valve they would not get any treatment,’ but there’s always the question if that is really the case.” If at all possible, devices should also be tested in Western countries, Kappetein urged.
Taking on TAVR
Data on the aortic valve, meanwhile, are still in the preclinical stage. Patrick W. Serruys, MD, PhD (Imperial College London, England), presented the first results for this indication at EuroPCR. According to Leon, the signs look positive: “The hemodynamics were very good. That is very encouraging—that there were as good [and] you could argue that they were even better than a control surgical bioprosthetic valve.”
Histopathology findings on the aortic device also showed that there is a “transition point” when the polymer shifts over to endogenous tissue, Leon said. “We’re still kind of learning when that transition point is, and it doesn’t seem to be the same for all parts of the valve; it may be that the hinge points are different from the valve leaflets.”
Notably, the Xeltis valves designed for use in the left side of the heart are strengthened by an underlying nitinol frame. But Kappetein reassured that since aortic valves would mainly be used in older patients who aren’t growing, the existence of the frame isn’t a limitation. “The advantage in elderly people would be you still have a leaflet that can regenerate, so it’s living tissue,” he said, adding, “It’s more natural. It’s your own tissue.”
Improving access to TAVR is still key, Leon said, noting that only 30% of adults with symptomatic aortic stenosis in the United States actually receive valves.
ETR has a number of other advantages over bioprosthetic valves, Leon pointed out to TCTMD, including the sheer amount of source material required. “It takes as many as 10 cows to get a single heart valve,” he said. There’s also challenges related to the source material itself, which has important differences from living human-valve tissue. With cow- and pig-based materials “the tensile consistency and chemical consistency is difficult [to control],” Leon noted, “We tend to process the material, so we fix it in glutaraldehyde. And glutaraldehyde induces an inflammatory reaction [in the body].”
With ETR, he suggested, “you avoid those early inflammatory responses, which may in fact be associated with thrombus formation” that’s suspected to be at the root of valve deterioration.
The Challenges Ahead
A big unknown is whether tissue-engineered devices will perform as well as hoped over the long term.
The potential for leaflet retraction—and its deleterious effect on durability—is one of the main issues to be addressed, Kappetein commented. “Leaflets can get thickened over time and then they can retract, and then you get a leaking heart valve.”
On the right side of the heart, valve retraction could result in pulmonary insufficiency. On the left side, when treating patients with stenotic, calcified valves, there’s the possibility of paravalvular leakage if the implant cannot be expanded until it’s completely circular. Circular expansion “is important, so that the three leaflets open in the same way [and] the forces on the leaflets are evenly distributed. This also has an impact on durability,” he explained. “There are a lot of issues that need to be solved before we can say this is really working.”
The scientific concept has to be solid and it has to work, because this is at the end what will translate into safety and also predictability for the patient. Maximilian Y. Emmert
Conventional valves can be tested ex vivo, Leon observed. “The frame we test for 600 million cycles, which is 15 years, and the valve we test for 5 years on an accelerated pulse duplicator where it’s beating much faster,” he said. “We can actually look at the valves in physiologic conditions and see if there’s any evidence of deterioration of the valve prematurely.”
But with a polymer that will eventually disappear, that’s impossible, he noted. “The actual valve that’s going to be [in the body] permanently is a valve that is basically being formed in situ.” One possibility, Leon suggested, would be to implant the resorbable devices in animals, wait until the endogenous valve forms and explant it, and then test the valve.
Similarly, Emmert stressed the need for basic research that could enable tissue engineering to cross into clinical practice. “The scientific concept has to be solid and it has to work, because this is at the end what will translate into safety and also predictability for the patient,” he said.
And one day, a long way down the path only now being forged will lie the question of just how regulatory agencies used to evaluating drugs and devices will categorize and label products of the future. Should they be classified as cell therapy? Are they devices? Are they many things at once? “Authorities base their decision to approve a product or a concept by comparing it to the experience they have with other devices. And here the challenge is there are no other devices yet,” Emmert said. In other words, we’re in uncharted territory.
Caitlin E. Cox is News Editor of TCTMD and Associate Director, Editorial Content at the Cardiovascular Research Foundation. She produces the…
Read Full BioDisclosures
- Cox reports being founder of and chief technology officer for Xeltis as well as being a shareholder in the company.
- Emmert reports no relevant conflicts of interest.
- Kappetein reports that his institution receives grant funding from the Dutch Heart Foundation to work on a tissue-engineered heart valve.
- Leon reports serving on the medical advisory board of Xeltis as an unpaid consultant.
- Serruys reports receiving grant/research support and consulting fees/honoraria with numerous companies including Xeltis.
Comments